Cardiovascular magnetic resonance (CMR) continues to provide exceptional quality images of cardiac and major vessel anatomy, but recent advances now permit a rapid, robust assessment of a diverse spectrum of functional and physiological parameters. In the routine CMR investigation of ischaemic heart disease, clinicians are now faced with an array of real time on-line rest and stress images that encompass cardiac function, perfusion, the presence of infarcted, viable and ischaemic myocardium, as well as metabolism.
The implementation of cardiac dedicated scanners, the ever increasing array of diagnostic information available, and the increasing clinical demand for all forms of CMR investigation has seen the integration of MRI into cardiology, with cardiologists becoming increasingly involved at every stage. With the advent of CMR directed intervention and CMR directed therapy, together with the development of combined cardiovascular interventional CMR laboratories, interventional cardiologists in particular now themselves in the forefront of this pioneering technology.
This article reviews the key areas of CMR that pertain to the modern interventional cardiologist.
Introduction
Cardiovascular magnetic resonance (CMR) is recognised as the gold standard, non-invasive investigation for the delineation of cardiac and major vessel anatomical variation, particularly in the setting of congenital heart disease (Figure 1).
Figure 1. Coarctation of aorta.
It provides images of exceptional precision and quality throughout a wide range of cardiovascular disease processes. Recent improvements in the technical capabilities and speed of acquisition of the scanners has sponsored a more robust role for CMR in the setting of coronary artery disease. CMR images can now be rapidly acquired and manipulated to give information on cardiovascular structure, function, metabolism, perfusion, as well as the presence of infarcted, viable and ischaemic myocardium.
With the development of dedicated CMR scanners as well as combined cardiovascular interventional CMR laboratories, interventional cardiologists are now an integral component in the evolution of this pioneering technology.
Basic principles of CMR
Magnetic resonance imaging produces images by intermittently exciting hydrogen nuclei (protons) using a magnet field and radiofrequency waves. In order to overcome artefacts produced by respiratory and cardiac motion ultra-fast sequences are used, together with ECG and sometimes respiratory gating.
Modern MRI magnets are shorter, more open and generally more comfortable for patients to lie in, and are equiped with cardiac specific software sequences to optimize cardiac imaging. Patients with implantable defibrillator/pacemakers and cerebral artery clips are not generally accepted for scanning but nearly all prosthetic valves and all coronary stents can be safely scanned. The patient is not exposed to any ionising radiation and there are no known side effects of MRI.
Many of the recent advances have been made possible by a dramatic increase in speed of acquisition. For example, a three dimensional rotating angiogram (Figure 2) can be acquired in 20 seconds, while a complete stack of short axis 16 frame cine slices encompassing the entire left ventricle can be acquired in a single breath hold.
Figure 2. CMR angiography of coarctation.
Assessment of cardiac function
Coronary artery disease is the leading cause of heart failure in the Western world. The degree of left ventricular dysfunction is an important prognostic indicator and a significant determinant of outcome following revascularization. An accurate and reproducible assessment of global and regional cardiac function is therefore essential.
CMR offers a precise, accurate and highly reproducible assessment of both left and right ventricular function that is free of any geometric assumptions by providing multi-frame moving images of the heart in any desired plane (Figure 3).
Figure 3. CMR provides a three dimensional reconstruction of the heart.
A contiguous stack of short axis cine images that encompass the entire ventricle can be acquired. The end diastolic and end systolic volumes (and thereby ejection fraction) can be calculated by the addition of the respective end diastolic and end systolic volume of each short axis slice. In this way the entire ventricular volume is included without the need for the geometric assumptions used by other techniques, such as those that only measure a single ventricular diameter and length and use a mathematical model that assumes the ventricle is a perfect cone shape.
The reproducibility is so much better than alternative imaging modalities that sample sizes for research studies can be dramatically reduced. For example, to detect a 3% difference in ejection fraction CMR requires a sample size of 15 compared to 102 by echo, an 85% reduction for a power of 90% and p<0.05. (Similarly, a 10g difference in left ventricular mass by CMR requires 9 patients compared with 273 for echo)1-3.
The high resolution movie images allow for quantitative regional wall motion analysis, with every segment clearly visible. Furthermore, the distortion of an imposed ‘tagging’ grid (Figure 4) during the cardiac cycle, or direct CMR tissue velocity mapping provides quantitative measurements of intrinsic myocardial mechanics.
Figure 4. Tagged short axis slice in diastole and systole.
Infarct imaging
Extensive animal and human studies have demonstrated that acute and chronic myocardial infarctions hyper-enhance on gadolinium contrast CMR images. Furthermore, the spatial extent of hyper-enhancement correlates closely with the spatial extent of histologically defined areas of myocardial necrosis4,5. This in vivo virtual histological imaging can be used to clearly define areas of full thickness (Figure 5) or sub-endocardial infarction that may be amenable to intervention (see viability section).
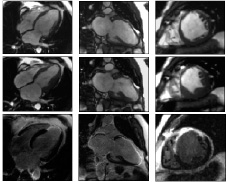
Figure 5. End diastolic, end systolic and delayed enhance CMR frames. An area of thin myocardium which fails to thicken can be seen in the distal septum, anterior wall and apex consistent with an anterior myocardial infarction. The delayed enhanced CMR defines areas of myonecrosis in white. This example demonstrates a full thickness MI that has no viable myocardium and would not improve with revascularization. This information can be obtained in the setting of an acute or chronic MI prior to considering PCI.
Not only does CMR define the degree and extent of infarction, it also differentiates areas that suffer from poor tissue perfusion following revascularization; the no-reflow phenomenon. This area of no-reflow represents severe microcirculatory damage, myocyte necrosis and localized oedema that compresses intramural vessels. This area, which can be seen as a central black core on delayed gadolinium scanning (Figure 6), has been shown to have no propensity for recovery and a strong predictor of adverse remodelling6.
Figure 6. Full thickness myocardial infarction of anterior wall (white gadolinium uptake) with central dark core of no-reflow following stent deployment in left anterior descending artery.
So dramatic is the spatial resolution of CMR that, for the first time, the direct in vivo consequence of percutaneous intervention (PCI) side-branch occlusion can be appreciated (Figure 7).
Figure 7a. PCI and stent (X) to left anterior descending artery, with compromise to and reduced flow in the diagonal branch.
Figure 7b. End diastolic, end systolic and delayed enhance CMR frames. This patient suffered a MI which was treated with thrombolysis. In view of ongoing angina they went on to have an angiogram and PCI to a significant LAD stenosis with a good angiographic LAD result. The LAD stent crossed the first diagonal branch with some nipping and TMIT II flow. An area of distal anterior wall motion abnormality can be seen corresponding to the anterior MI. The DE CMR in this region reveals small patches areas of full thickness myonecrosis (white) but a significant degree of only sub-endocardial myonecrosis. Viable myocardium is therefore present within this infart zone, and the patient will benefit from PCI. This study illustrates the high resolution of this technique as the basal short axis slice reveals a small diagonal branch during PCI. Note that this damage is too small to cause a regional wall motion abnormality.
Thus CMR further extends our understanding by providing an anatomical correlate to biochemical evidence (mild CK rise) of procedure-related myocardial injury that is often too small to produce an ECG change or discrete wall motion abnormality7.
Viability
Perhaps the most important recent CMR development for the interventionalist is the precise delineation of viable myocardium by gadolinium enhanced CMR (Figure 5, 6 and 7).
Patients with ischaemic, but viable myocardium who are successfully revascularized have more than a four-fold decrease in annual mortality when compared to those treated medically8. Furthermore, medically treated viable myocardium is the harbinger of further non-fatal ischaemic events and significant morbidity9. For example, in a recent randomised trial of late PCI (3.6 weeks) to an isolated occluded left anterior descending artery versus medical therapy, there was a significant improvement in left ventricular remodelling and function at one year in those patients who underwent PCI and had viable myocardium. By contrast, PCI patients without viable myocardium, as well as the group receiving medical therapy alone, failed to show any beneficial remodelling10.
Conversely, those patients who undergo revascularization who do not have viable myocardium are exposed to significant risk with little benefit (particularly if a surgical option is preferred, especially as these patients frequently have severe left ventricular dysfunction). This is highlighted in a recent meta-analysis which suggests the annual mortality rate following revascularization is twice as great in patients without viable myocardium, with a significant increase in peri-operative mortality (approx 10%) in the absence of viability9.
Knowledge of the presence or absence of viability is, therefore, essential to formulate an informed, evidence based revascularization strategy.
Gadolinium enhanced CMR provides exquisite differentiation of infarcted and viable myocardium without the need for the stress agents used by echo, and with greater resolution than PET and SPECT11, without exposure to ionising radiation. Robust, reliable sequences have been developed with image acquisition occurring in a single breath hold 15 minutes after contrast injection.
In a similar protocol to that employed by stress echo, stress CMR can also be performed to quantify regional contractile improvement with low dose dobutamine (with similar sensitivity and specificity to both PET and transoesophageal stress echo12,13). This assessment of viability, however, has been largely superseded by the reliable and rapid gadolinium enhanced imaging at rest.
Ischaemia
In coronary artery disease, revascularization decisions should ideally be based on both coronary artery stenosis anatomy, the haemodynamic consequence of this stenosis and the presence of distal viable myocardium.
Ventricular function, and presence of infarction and viability can be measured at rest by CMR, but inducible ischaemia can only be detected under stress conditions. The earliest sign of myocardial ischaemia is wall motion abnormality, and this occurs much earlier than angina symptoms or ECG changes. Alternatively, myocardial perfusion can be used to assess iscahemia.
Nuclear medicine (such as SPECT) assessment of ischaemia is severely limited by poor spatial resolution (it cannot, for example, differentiate sub-endocardial perfusion defects), attenuation artefacts, radiation exposure and local experience. Alternative techniques are required.
Wall motion stress CMR
Stress conditions can be reproduced pharmacologically using either dobutamine or adenosine. Both agents have been shown to be safe and well tolerated, but dobutamine at high dose (40 µg/kg plus atropine to sub-maximal heart rate) has higher reproducibility, more closely mimics the physiology of exercise, and is easier to control. Adenosine stress is associated with fewer side effects but has lower sensitivity than dobutamine.
Although gadolinium enhanced CMR is largely replacing wall motion analysis of viability, an ischaemic stress test proceeds to higher stress levels through the lower doses that can be used to assess viability in the same study session (Figure 8).
Figure 8. One of a stack of short axis slices at rest and increasing doses of dobutamine illustrating increased contraction with increasing dose.
The stress echo data using dobutamine in patients with suspected coronary artery disease have shown sensitivities and specificities within the ranges of 54-96% and 60-100% respectively14. This is dependant on the experience of the centre and is limited by a 10-15% non-diagnostic results14, poor specificities for basal-lateral and basal-inferior segments of the LV, and poor reproducibility of the test results15. The innate image quality of CMR not surprisingly means that even those patients with poor echo images can be easily assessed by CMR16. Furthermore, direct comparisons of transoesophageal stress echo (TOE) and stress CMR with subsequent cardiac catheterization in 208 patients with suspected coronary artery disease yielded significant improvements in sensitivity (86% CMR vs 74% TOE), specificity (86% CMR vs 70% TOE), and diagnostic accuracy (86% CMR vs 73% TOE) when using CMR17.
Stress testing is easily performed with the patient in a magnet, and can be completed in less then 30 min using three short axis and two long axis cine images to encompass the entire 17 segment AHA/ACC model18. The specialist involved needs to be aware of the effect of the magnetic field on the ECG trace, view and interpret the on-line images as the wall motion abnormality arises and be in a position to terminate the scan. In the unlikely event of a problem they will need to be able to treat (and resuscitate) the patient, much like stress echo labs that are already fully incorporated into the cardiology environment. Currently, few cardiologists are trained in stress echo, and even fewer in stress CMR. Local practice will inevitably depend on local facilities and experience, while specialist centres with both facilities will be able to direct their echo and CMR resources efficiently and tailor their investigations to best suit individual patients.
Perfusion
A large evidence base has built up for the prognostic significance of coronary artery disease by non-invasive methods19. These methods are based on the extent and severity of perfusion defects during exercise or pharmacological induced hyperemia. The ability of CMR to specifically differentiate the extent of ischaemic, viable (be that stunned or hibernating), and infarcted myocardium holds the promise of a more powerful prognostic tool.
As well as providing prognostic information, CMR can provide information regarding the effect of individual coronary artery stenoses, thereby tailoring revascularization strategies.
Perfusion CMR (Figure 9) utilizes a high spatial and temporal resolution to provide an accurate assessment of perfusion defects under maximum hyperemia.
Figure 9. Rest and stress perfusion for multiple short axis slices. As the white gadolinium contrast agent perfuses into the black myocardium, an area of inferior stress induced ischaemia shows up as a sub-endocardial black rim.
Although these high resolution images now allow the visual detection of even sub-endocardial defects, a further challenge is to provide true quantification. Much research is centred on this question and specific techniques have been developed. Multicentre trials are required to confirm the accuracy and robustness of these techniques. This information can then be combined with functional assessment and quantification of infarcted and viable myocardium, all achieved in the same CMR session. Such a comprehensive analysis will represent a powerful risk stratification model and help guide informed, appropriate coronary revascularization.
Coronary arteries
Invasive coronary angiography remains the gold standard for the diagnosis of obstructive coronary disease, and offers the potential for concurrent intervention. The search for an alternative non-invasive, radiation free CMR coronary angiography continues, with substantial improvements being made each year (Figure 10).
Figure 10. Maximum intensity projection images reconstructed from the 3D MR coronary angiograms (sequence parameters: TR 5.7ms, TE 2.8ms, flip angle 110°, matrix 272x272, reconstructed to an image resolution of 0.54x0.54x1.5mm). A) Oblique sagittal view demonstrating an anomalous origin of the left circumflex (arrowhead) from the RCA (arrow). B) Coronal view demonstrating an anomalous accessory left anterior descending artery (arrowhead) arising from the same ostia as the RCA (arrow). No ostial stenoses or proximal coronary artery kinking are seen.
Copyright owned by Taylor AM. Printed with permission. Original publication: Taylor AM, Dymarkowski S, Hamaekers P, Razavi R, Gewillig M, Mertens L, Bogaert J. Magnetic resonance coronary angiography and late-enhancement myocardial imaging in children with arterial switch operation for transposition of the great arteries. Radiology 2005; 234:542-547
With current CMR techniques it is possible to consistently image proximal and middle portions of coronary arteries with a spatial resolution of 1.5mm3, and CMR has already been shown to have a negative predictive value which may be useful for ruling out significant left main and proximal coronary artery disease20. CMR remains ideally suited to the depiction of anomalous coronary arteries and their relation to surrounding structures, the aortic root and pulmonary trunk for example21. Furthermore, the relative large diameter and limited motion of vein grafts make thses structures relatively easy to image.
In terms of imaging the entire native coronary artery bed, the role of CMR remains limited. Ongoing research aimed at improving spatial resolution and overcoming cardiac and respiratory motion artefacts mean that CMR may yet become an important tool in the assessment of coronary artery stenoses, particularly when combined with the morphological, functional, haemodynamic and metabolic information this technique affords. At present, however, CT holds more promise for reliable and consistent imaging of coronary arteries, albeit with significant radiation exposure. With the advent of 16 row multi-slice CT, the sensitivity and specificity for detection of stenoses > 50% is approaching 90%, with the aid of beta-blockers to keep the heart rate <60 beats per min (patients have to hold their breath for 20 seconds)22,23. Limitations still exist, however, in calcified arteries and beam hardening artifacts related to stent materials limit visualization of stent lumen. Furthermore, the poorer temporal resolution compared with MRI makes it less suitable for functional and wall motion assessment.
Atherothrombotic plaque imaging
Imaging of plaques in coronary arteries is complecated by the small size of the arteries, their tortuous course, low signal to noise ratio between the arteries and adjacent fat, as well as cardiac and respiratory motion. Work, therfore, started on aortic and peripheral artery plaques where the extent of atherosclerotic plaque can be demonstrating as well as the fissuring, local dissection and later remodelling following balloon angioplasty24,25. Research now centres on applying these principles to the challenge of coronary arteries with encouraging initial results26. In terms of plaque composition, it is not yet however possible to characterize plaque components due to overlapping signals between the lipid core and vessel media, particularly in the more subtle vulnerable lesions. Techniques such as the use of intravascular contrast agents and iron labeled macrophages are being developed to overcome this and help identify vulnerable plaques27,28.
Interventional CMR
The X-ray fluoroscopy used in current cardiac catheterisation labs provides excellent spatial and temporal resolution, but soft tissues are virtually impossible to distinguish and the radiation dosage is potentially harmful to patients and staff. Attempts to overcome poor tissue characterization has see the use of ultrasound during interventional procedures, but the image quality is often limited by the ‘ultrasound window’. CMR offers the potential benefits of soft tissue visualization in any plane without the use of ionising radiation. As a result, interventional CMR labs have now been set up to initially complement these other modalities, as well as allow research and development to further expand the role of CMR (Figure 11).
Figure 11. Schematic room plan of an XMR facility. b,c) Photographs of the room view from b) the side, and c) the end of the X-ray table. The C-arm is seen in the foreground (arrowhead), the ceiling-mounted MR monitor and controls are seen in the distance (arrow), and the 5-gauss colour marking in shown (asterisk). Image from: Clinical Cardiac MRI (with Interactive CD-Rom), Edited by Bogaert J, Dymarkowski S, Taylor AM. Springer-Verlag, Berlin Heidelberg, 2005;1-549. Fig. 17.6 ISBN 3-540-40170-9. With kind permission of Spinger Science and Business Media and Andrew M Taylor.
Early experience
Early experience centred on the use of CMR for initial assessment of anatomical and lesion characteristics, as well as vascular flow in the angioplasty of aortic coarctation and carotid artery stenosis. The angioplasty was then performed (in the combined lab) using conventional XRF, with an immediate post angioplasty CMR to assess the result and modify further intervention.
Endovascular angioplasty and stent placement
Improvements in MRI hardware, the development of internal receiver coils, real time imaging/tracking and MR compatible stents (nitinol and tantalum) have made it attractive to position peripheral and coronary stents using CMR guidance. CMR not only allow precise stent placement but also affords information of plaque composition and blood flow characteristics.
Following considerable animal work29, Manke et al.30 described the first human MR guided iliac stent placement in 13 patients using gadolinium solution to inflate the angioplasty balloon. Since this early work, numerous reports have been published of successful MR guided stent placement in major arteries and veins of animals. Spuentrup et al, for example, have developed a swine coronary artery model and have successfully implanted intra-coronary stents31. This has provided some proof of concept, although many technical and safety issues still need to be addressed in the race to provide a reliable, robust technique. For example, there are two main methods of visualizing the guide catheter, passive and active. The former uses the properties of the guide catheter, or the fluid within it, to allow visualization. Most catheters used in the cath lab, however, are metal braided and therefore have the potential to move in the MR magnetic field, heat up or induce a current. The same is true for the guide wires (even the nitinol ones). The lack of approved MR compatible guide catheter and guide wire material prohibits its widespread clinical use. The second method of visualizing the guide is active. These catheters contain a signal coil either at the tip or along its length to allow accurate localization. This was first described by Leung32 and has been used successfully in targeted intra-myocardial stem cell injection in animals33. This method holds much promise, but safety issues once again limit its use as the coils that are inevitably connected to the external circuits can induce electrical currents and are susceptible to heating. Further technical innervations, such as using fibre optic connections and even using the stent itself as in internal receiver coil are currently in development.
We are, therefore, currently at the stage of using both X-ray angiography and CMR to guide therapy, as a step towards CMR intervention. The limitations of MR compatible guide catheters, guide wires and stents mean that PCI and arrhythmia ablations are currently performed using X-ray guidance, but in combined X-ray/CMR labs the CMR provides complementary anatomical information as the patient moves seamlessly from CMR to x-ray imaging.
Clearly there is a way to go yet before we see the routine use of CMR guided angioplasty, but given the rate at which CMR technology has developed it is not likely to be long before the interventional cardiologist will be expected to adapt their skills to the interventional CMR lab.
ASD closure
In vivo animal studies have demonstrated that catheter tracking and ASD device closure can be performed under real-time MRI guidance with the use of intravascular antenna guide wires. For example, 7 anaesthetized pigs with defects of the atrial septum were catheterized using venous and arterial access by Rickers et al34. High-resolution imaging allowed accurate determination of ASD size before the intervention. A prototype active tracking catheter was used to obtain blood pressures and samples from cardiac chambers and great vessels using antegrade, transseptal, and retrograde approaches. Real time CMR fluoroscopy was used to locate and steer the catheter, while velocity-encoded cine MRI to measure pulmonary and aortic blood flow and calculate vascular resistances before and following closure. This work is encouraging although, as previously described, the guide wire used can not be safely used in patients.
Transcatheter valve stents
Transcatheter placement of valved stents for the treatment of pulmonary regurgitation has been validated in animal models35. Self expanding nitinol stent valves were deployed over a nitinol guidewire (this wire is not safe in patients) in the pulmonary artery under real-time CMR tissue guidance. Subsequently, phase contrast CMR was used to assess flow volumes within and distal to the valve. This procedure is limited by sub-optimal stent lumen visualization even when using nitinol, although this can be significantly improved by the use of gadolinium contrast.
Therapeutic myocardial injection
Two main areas have demonstrated potential benefit from myocardial injection. The first is the injection of stem cells into areas of myocardial infarction in the hope of regenerating heart muscle36. The second, gene therapy, holds potential for the treatment of atherosclerotic cardiovascular diseases. Preclinical trials have demonstrated that genes can induce the growth of new blood vessels (angiogenesis) which could represent a major impact in the treatment of ischaemic heart disease, particularly in the setting of infarcted myocardium, occluded coronary arteries not amenable to PCI, in-stent restenosis, and previous CABG or co-morbidity restricting further intervention. Furthermore, genes have been shown to stabilize plaques, reduce plaque progression and the risk of rupture, as well as correct the morphological balance of low and high density lipoproteins and regenerate myocardial cells themselves.
The challenge remains the precise delivery of genes to the targeted atherosclerotic plaques or affected myocardium, with subsequent transfer of genes into the target cells for correct gene expression. The role of the interventionalist in this process is likely to become a key factor, as catheter based direct delivery holds the promise of precise local delivery with minimal systemic affects, and avoids the limitations of injection down occluded intra-coronary arteries.
CMR has a number of advantages over traditional XR fluoroscopy for the delivery of genes. As well as providing precise tissue characterization, a gadolinium/gene vector medium can be used to directly visualize the site of gene delivery and local tissue uptake. Once in the tissues, gene transfection and expression is required, a process that is significantly enhances by local heat delivery by activation of heat shock proteins and increased cell membrane permeability. The interventional guide wire used in CMR systems has been adapted to act not only as a conventional guide wire, but also as an internal receiver coil for enhanced image quality with local intra-vascular heating for effective transfection37. Similarly, a stem cells labelled with iron oxide enable direct visualization of site of injection, and serial follow up38,39.
In the identification of the vulnerable plaque, the development of a variety of specific site targeted MR contrast agents means it is now possible to produce MR enhancement in vulnerable plaques (by fibrin specific nanoparticles that target the presence of microthrombi)40, as well as areas of apoptosis (using a C2-glutathiones-transferase fusion protein)41. After successful catheter based delivery of the genes, and MR enhanced transfection, the final gene expression can be imaged using MR with the aid of imaging marker genes that encode for enzymes that modify pro-drugs, or cell-surface ligand binding proteins that accept MR specific imaging tracers. Ultimately, increased angiogenesis and relief of ischaemic burden can be accurately quantified by MR perfusion techniques.
Research continues in this area and it remains to be seen if CMR guided therapeutic injection is a safe or realistic prospect.
Conclusion
CMR continues to provide exceptional quality images of cardiac and major vessel anatomy, but recent advances now permit a rapid, robust assessment of a diverse spectrum of functional and physiological parameters. In the routine CMR investigation of ischaemic heart disease, clinicians are now faced with an array of real time on-line rest and stress images that encompass cardiac function, perfusion, the presence of infarcted, viable and ischaemic myocardium, as well as metabolism (Figure 12).
Figure 12. Summary of typical scan sequences.
Which of these diverse CMR tools are most appropriate for an individual patient, increasingly depend on clinical decisions made during the actual scan. The whole of dynamic CMR imaging is now so diverse and so fundamentally different to axial cross-sectional imaging that a new breed of CMR specialists and specialist units are emerging. The implementation of cardiac dedicated scanners, the ever increasing array of diagnostic information available, and the increasing clinical demand for all forms of cardiac MR investigation has seen the integration of MRI into cardiology, with cardiologists becoming involved with each patient specific CMR scanning sequence. With the advent of CMR directed intervention and CMR directed therapy, together with the development of combined cardiovascular interventional CMR laboratories, interventional cardiologists in particular now find themselves in the forefront of this pioneering technology. The successful CMR unit requires adult and paediatric cardiologists, interventional cardiologists and cardiothoracic radiologists who have extensive and specific CMR training to work closely together, integrating their own particular skill base.